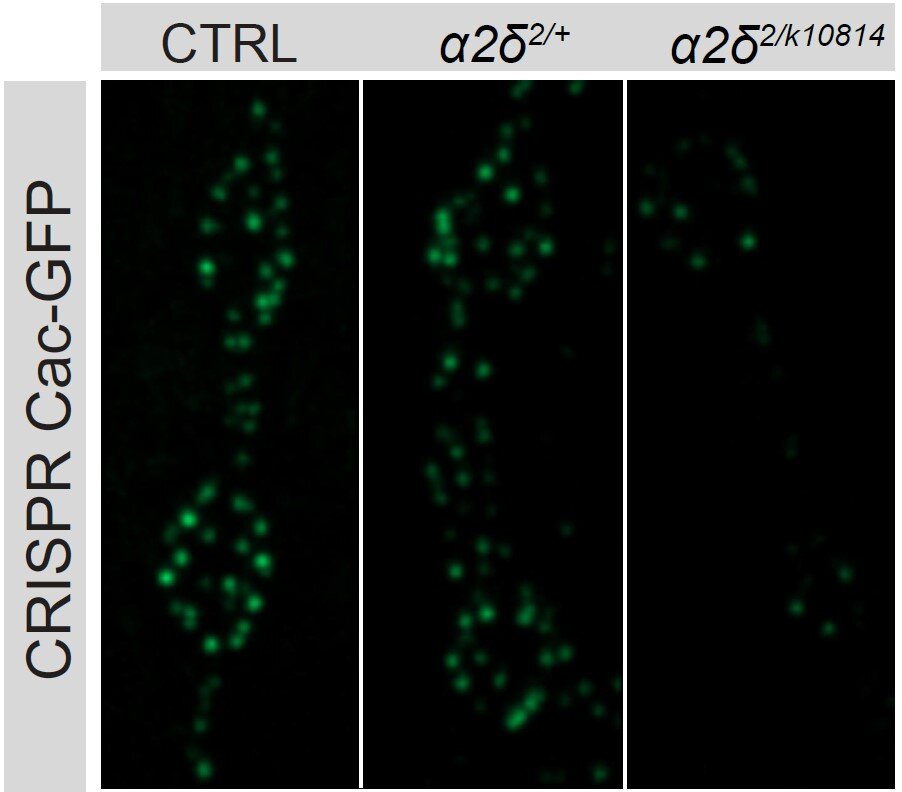
The nervous system works because neurons communicate across connections called synapses. They “talk” when calcium ions flow through channels into “active zones” that are loaded with vesicles carrying molecular messages. The electrically charged calcium causes vesicles to “fuse” to the outer membrane of presynaptic neurons, releasing their communicative chemical cargo to the postsynaptic cell. In a new study, scientists at The Picower Institute for Learning and Memory at MIT provide several revelations about how neurons set up and sustain this vital infrastructure.
“Calcium channels are the major determinant of calcium influx, which then triggers vesicle fusion, so it is a critical component of the engine on the presynaptic side that converts electrical signals to chemical synaptic transmission,” said Troy Littleton, senior author of the new study in eLife and Menicon Professor of Neuroscience in MIT’s Departments of Biology and Brain and Cognitive Sciences. “How they accumulate at active zones was really unclear. Our study reveals clues into how active zones accumulate and regulate the abundance of calcium channels.”
Neuroscientists have wanted these clues. One reason is that understanding this process can help reveal how neurons change how they communicate, an ability called “plasticity” that underlies learning and memory and other important brain functions. Another is that drugs such as gabapentin, which treats conditions as diverse as epilepsy, anxiety and nerve pain, binds a protein called alpha2delta that is closely associated with calcium channels. By revealing more about alpha2delta’s exact function, the study better explains what those treatments affect.
“Modulation of the function of presynaptic calcium channels is known to have very important clinical effects,” Littleton said. “Understanding the baseline of how these channels are regulated is really important.”
MIT postdoc Karen Cunningham led the study, which was her doctoral thesis work in Littleton’s lab. Using the model system of fruit fly motor neurons, she employed a wide variety of techniques and experiments to show for the first time the step by step process that accounts for the distribution and upkeep of calcium channels at active zones.
A cap on Cac
Cunningham’s first question was whether calcium channels are necessary for active zones to develop in larvae. The fly calcium channel gene (called “cacophony,” or Cac) is so important, flies literally can’t live without it. So rather than knocking out Cac across the fly, Cunningham used a technique to knock it out in just one population of neurons. By doing so, she was able to show that even without Cac, active zones grow and mature normally.
Using another technique that artificially prolongs the larval stage of the fly she was also able to see that given extra time the active zone will continue to build up its structure with a protein called BRP, but that Cac accumulation ceases after the normal six days. Cunningham also found that moderate increases of decreases in the supply of available Cac in the neuron did not affect how much Cac ended up at each active zone. Even more curious, she found that while Cac amount did scale with each active zone’s size, it barely budged if she took away a lot of the BRP in the active zone. Indeed, for each active zone, the neuron seemed to enforce a consistent cap on the amount of Cac present.
“It was revealing that the neuron had very different rules for the structural proteins at the active zone like BRP that continued to accumulate over time, versus the calcium channel that was tightly regulated and had its abundance capped,” Cunningham said.
Regular refresh
The findings showed there must be factors other than Cac supply or changes in BRP that regulate Cac levels so tightly. Cunningham turned to alpha2delta. When she genetically manipulated how much of that was expressed, she found that alpha2delta levels directly determined how much Cac accumulated at active zones.
In further experiments, Cunningham was also able to show that alpha2delta’s ability to maintain Cac levels depended on the neuron’s overall Cac supply. That finding suggested that rather than controlling Cac amount at active zones by stabilizing it, alpha 2delta likely functioned upstream, during Cac trafficking, to supply and resupply Cac to active zones.
Cunningham used two different techniques to watch that resupply happen, producing measurements of its extent and its timing. She chose a moment after a few days of development to image active zones and measure Cac abundance to ascertain the landscape. The she bleached out that Cac fluorescence to erase it. After 24 hours, she visualized Cac fluorescence anew to highlight only the new Cac that was delivered to active zones over that 24 hours. She saw that over that day there was Cac delivery across virtually all active zones, but that one day’s work was indeed only a fraction compared to what had built up over several days before. Moreover, she could see that the larger active zones accrued more Cac than smaller ones. And in flies with mutated alpha2delta, there was very little new Cac delivery at all.
If Cac channels were indeed constantly being resupplied, then Cunningham wanted to know at what pace Cac channels are removed from active zones. To determine that, she used a staining technology with a photoconvertible protein called Maple tagged to the Cac protein that allowed her to change the color with a flash of light at the time of her choosing. That way she could first see how much Cac accumulated by a certain time (shown in green) and then flash the light to turn that Cac red. When she checked back five days later, about 30 percent of the red Cac had been replaced with new green Cac, suggesting 30 percent turnover. When she reduced Cac delivery levels by mutating alpha2 delta or reducing Cac biosynthesis, Cac turnover stopped. That means a significant amount of Cac is turned over each day at active zones and that the turnover is prompted by new Cac delivery.
Source: Read Full Article